Factors that affect the reaction rate
The reaction rate can be affected in many different ways. The reaction rate is changed to gain some kind of benefit, like speeding up cooking or improving the shelf life of food, making a pharmaceutical more effective or slowing down the progress of a disease, or giving a battery a longer life cycle or improving its efficiency. Here are some factors that affect the reaction rate:
- the chemical structure of substances
- the state of substances
- steric hindrances
- temperature
- concentration
- pressure
- area
- catalysts
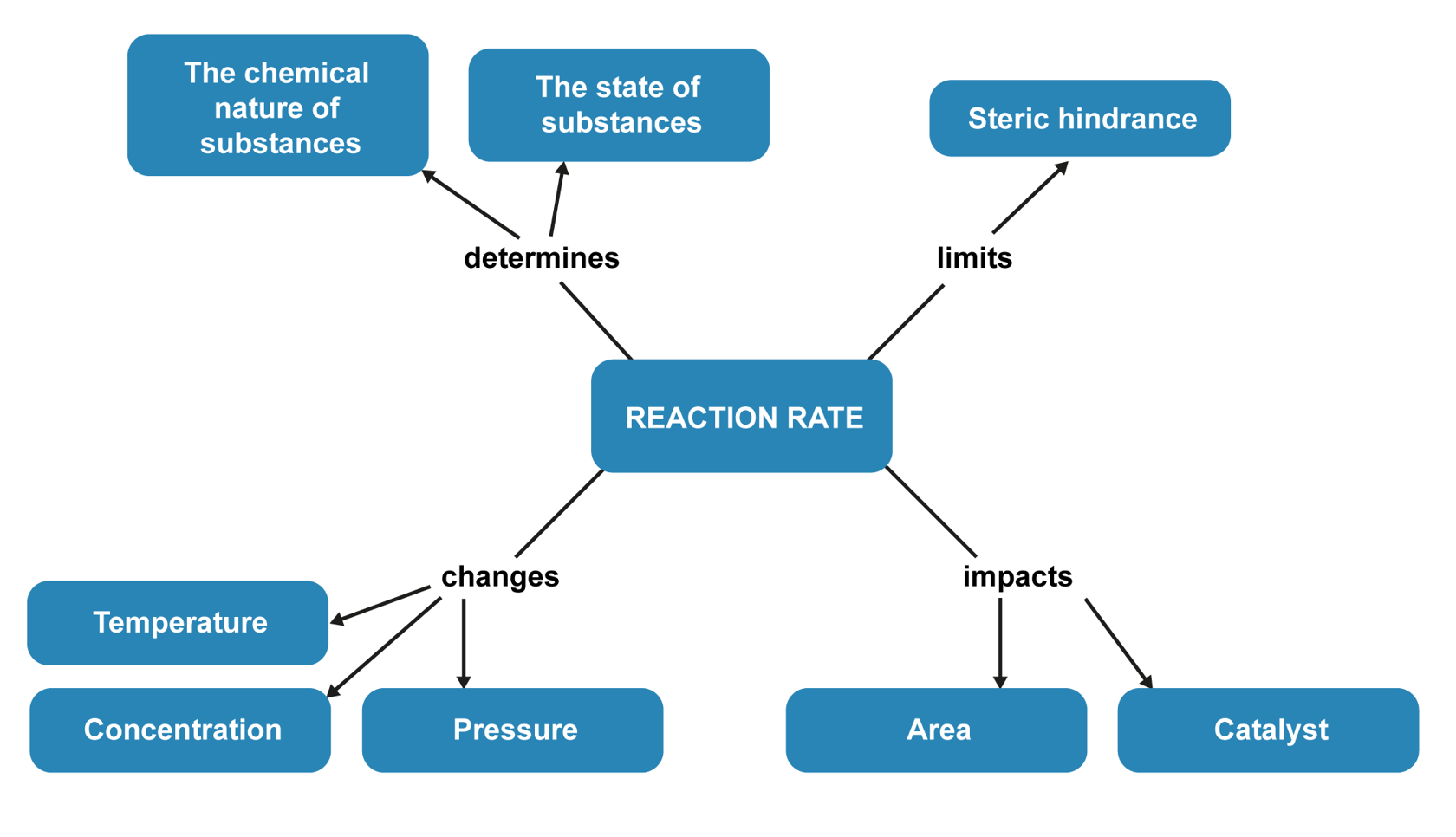
The collision theory
Two of the most common chemical reaction mechanisms are decomposition reactions that involve a single substance and collision reactions that involve two substances. There are also rarer collisions between three objects. Reaction mechanisms are referred to as uni- bi- and termolecular (trimolecular) reactions based on the number of reacting objects.
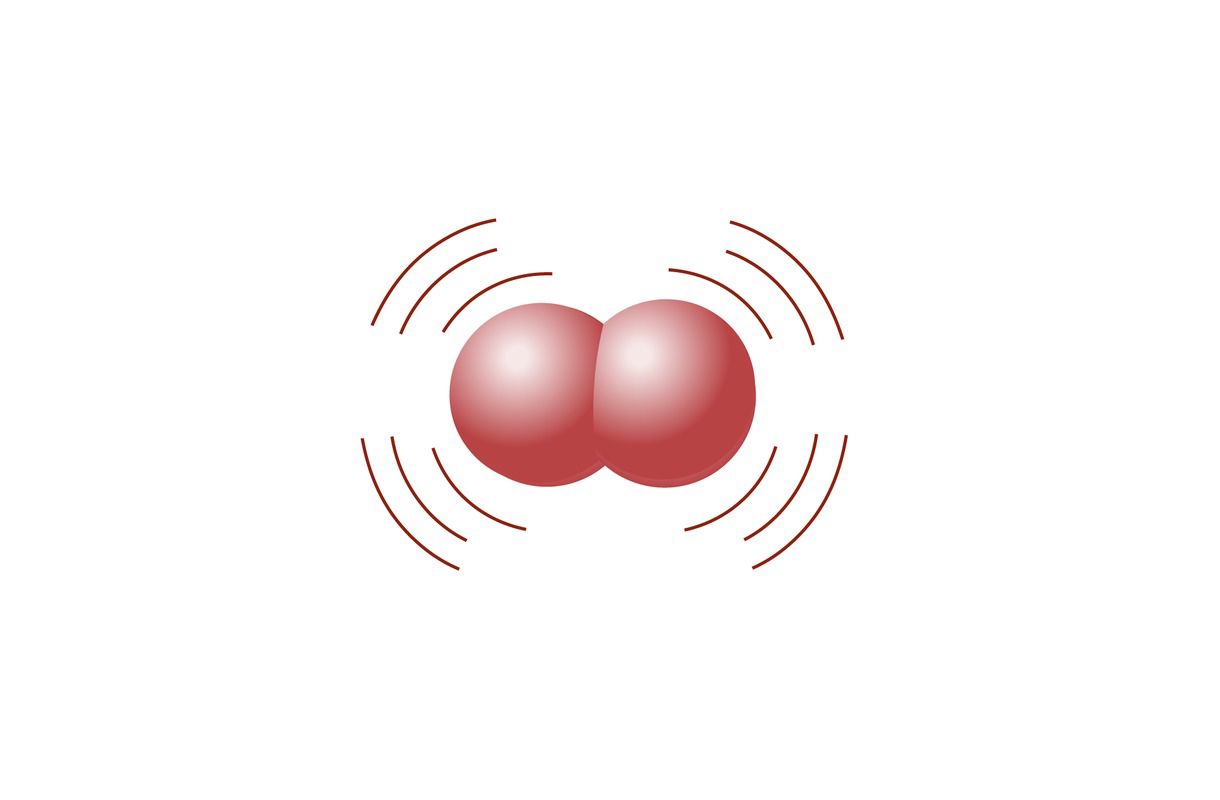
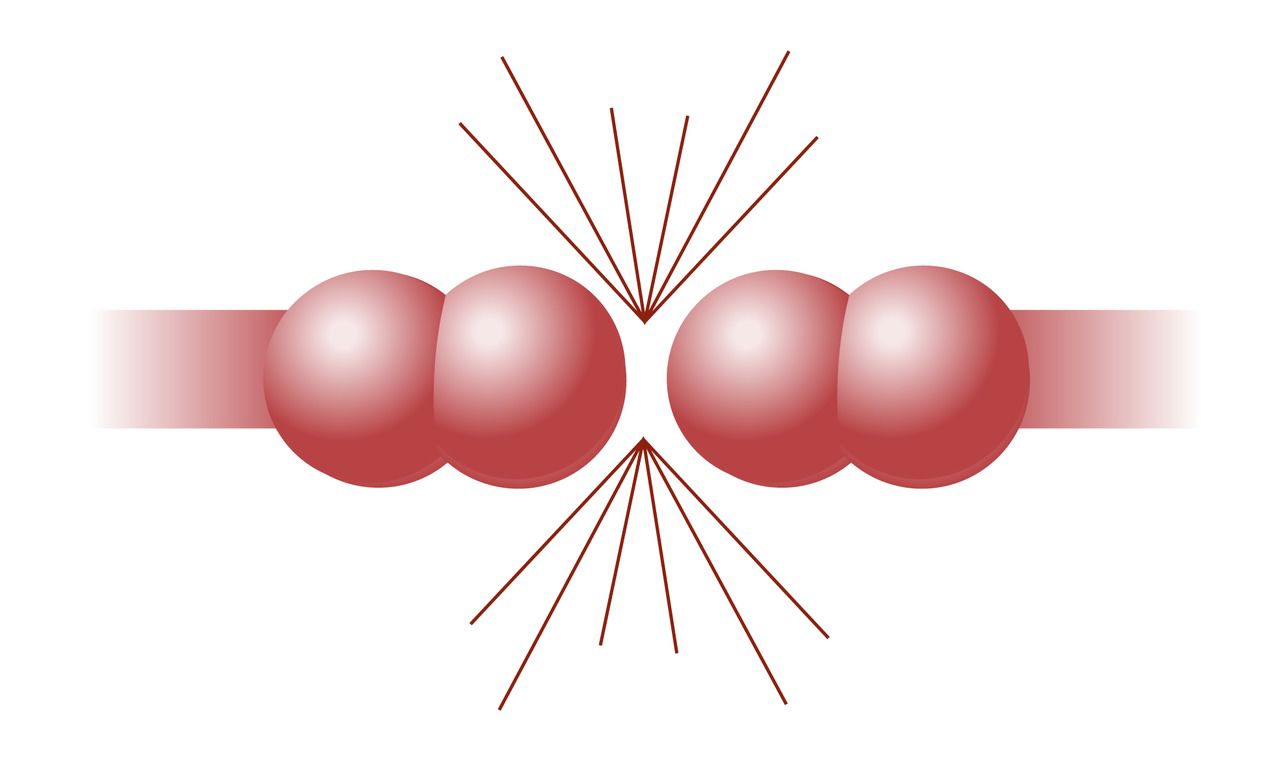
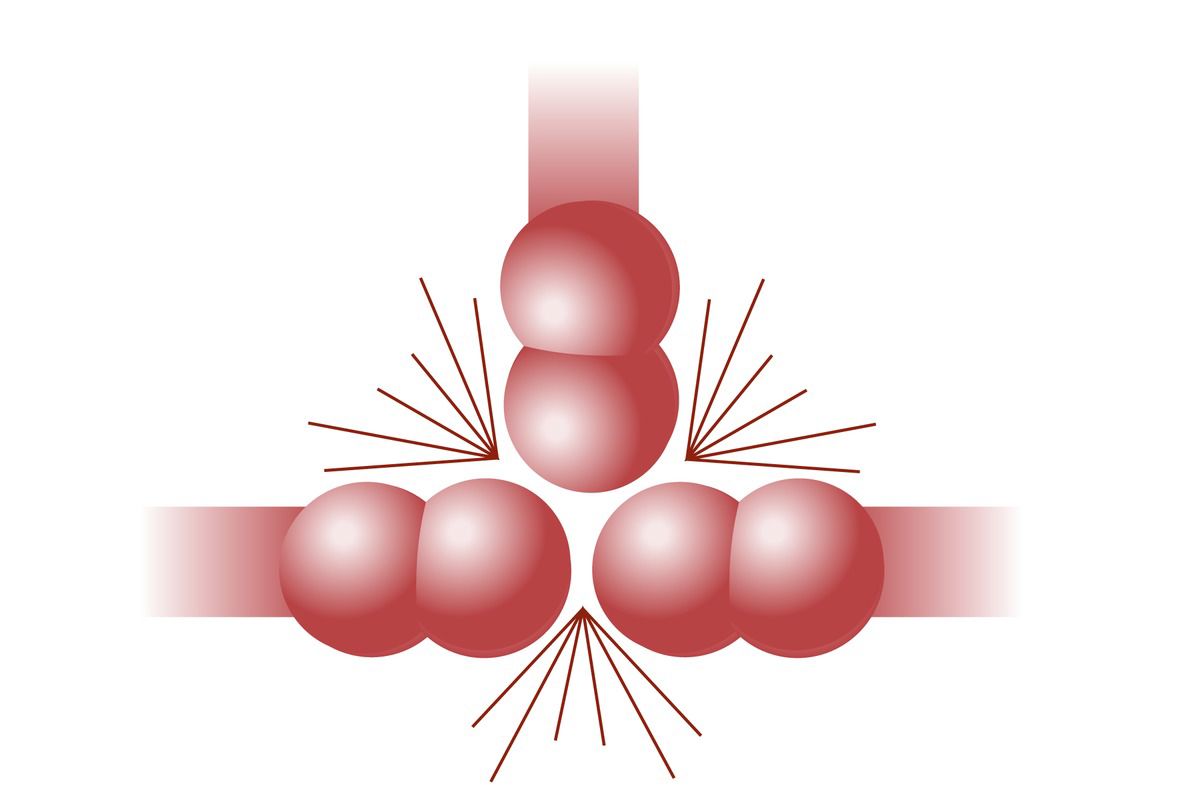
According to the collision theory, particles must collide at the right angle with enough kinetic energy to exceed the activation energy threshold. If the speed is too low or if the angle is wrong, the particles bounce off each other in different directions, and there is no reaction. In the graph below, the particles in the first reaction have enough speed, but the molecules have the wrong orientation, so no reaction occurs. The particles in the middle reaction have the right orientation, but they do not have enough speed, so there is no reaction. Only the last reaction in the image results in a reaction, because the particles have enough speed and the right orientation.
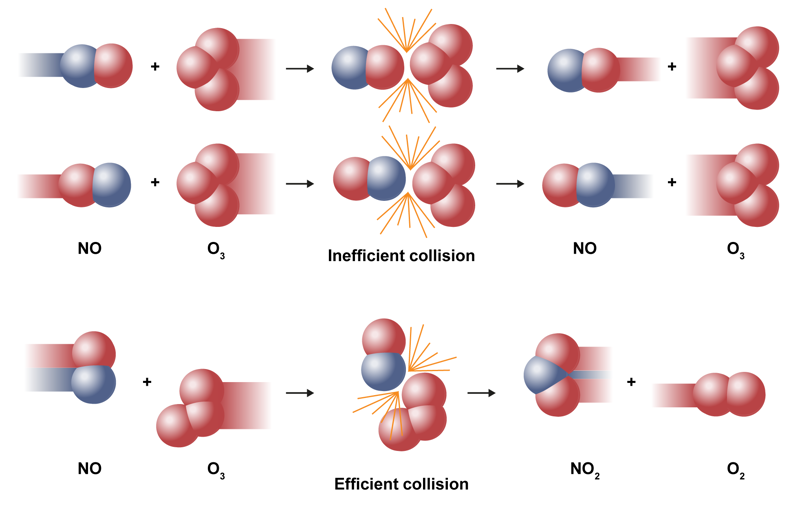
How the chemical nature of substances affects the reaction rate
A substance’s chemical consistency and atomic structure determine how easily substances will react with each other. Substances usually react with each other more easily if they are further apart in the periodic table.
When it comes to the main groups, metal reactivity increases when moving from the top of a group to the bottom. This is caused by the increase in atom size. The electric attractive force between the nucleus and electrons weakens as the distance increases, and electron separation becomes easier. For non-metals, the situation is reversed. Their reactivity decreases when moving from the top to the bottom. As the atom size increases, the nucleus’s attractive force for receiving electrons weakens, and reactivity decreases. When looking at elements in the same period, an element’s ability to receive electrons increases when moving from left to right. The substances in main group 1 donate electrons more easily, while the substances in main group 7 receive electrons more easily. The reactivity of other elements falls somewhere between the two extremes. Noble gases barely react with any substance, and their reaction rates are low.
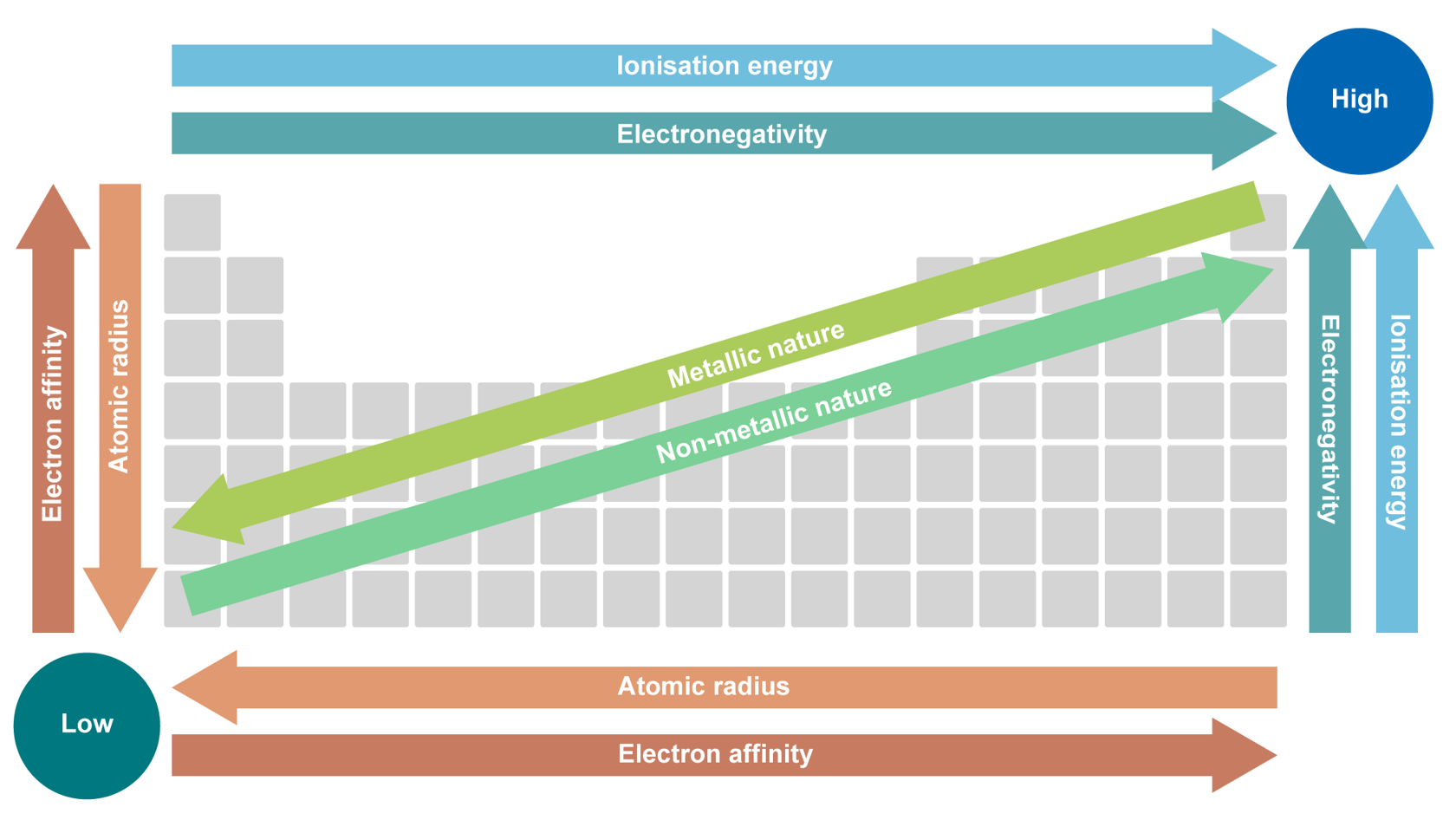
A substance’s chemical consistency and state affect reactivity. For example, gaseous nitrogen is very passive, whereas metallic iron reacts easily, even with oxygen. The ring structures in organic compounds are usually more passive than straight-chain structures. The active parts (multiple bonds and functional groups) in the ring or straight chain have a significant impact on substance reactivity and reaction rates.
Ion and radical reactions are usually very fast. Practically every collision results in a reaction, and the reaction rate is astoundingly high. Elements, compounds and molecules commonly have lower reaction rates.
How a substance’s state affects the reaction rate
Chemical reactions occur for all states – gases, liquids and solids. Reactions generally occur more quickly in gaseous phases and more slowly in solid phases. Liquid reactions occur at a rate somewhere between the gaseous and solid phase.
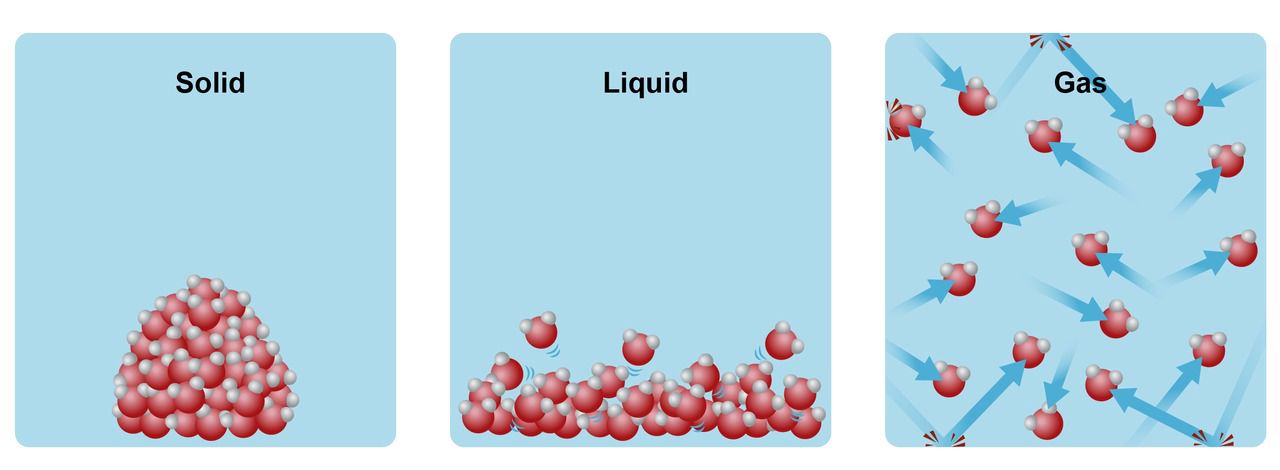
The significance of solvents varies. Studies done on dinitrogen pentoxide N2O5 for example, show that solvent nitrogen or decomposition in a liquid or gaseous phase do not affect decomposition reaction rates. On the other hand, solvents do have a significant impact on reaction rates in some organic reactions.
Organic compounds usually have high reaction rates with polar solvents, such as water, alcohol or ketone. In contrast, the reaction rates are low when using nonpolar solvents, like hydrocarbons or ether. The factors that determine a solvent’s impact on the reaction rate are viscosity, polarity and changes in the reacting molecules’ bond strength caused by hydration. The impact is not really determined by changes in the number of colliding particles. For example, hydration usually means a higher degree of order, which results in reduced entropy and also a lower activation energy level.
Many reactions occur between substances in different states. When a metal dissolves into an acid, the reaction occurs on the solid substance and liquid’s interface. When a metal oxidises in open air, the reaction occurs on the solid substance and gas’s interface. Reactions also occur between substances in different states in many biochemical processes, such as digestion, breathing and aging. Activated carbon filters absorb liquid or gaseous contaminants to the surface of solid carbon. Phenomena like this have three distinct stages: 1) the reactive substances enter the reaction zone, 2) the reaction occurs, 3) the reaction products exit the reaction zone. If the reaction product does not exit the reaction zone, a passive layer may be formed. This happens when aluminium is coated with a protective oxide layer, for example. The protective layer has a significant impact on the reaction rate and can prevent further reactions.
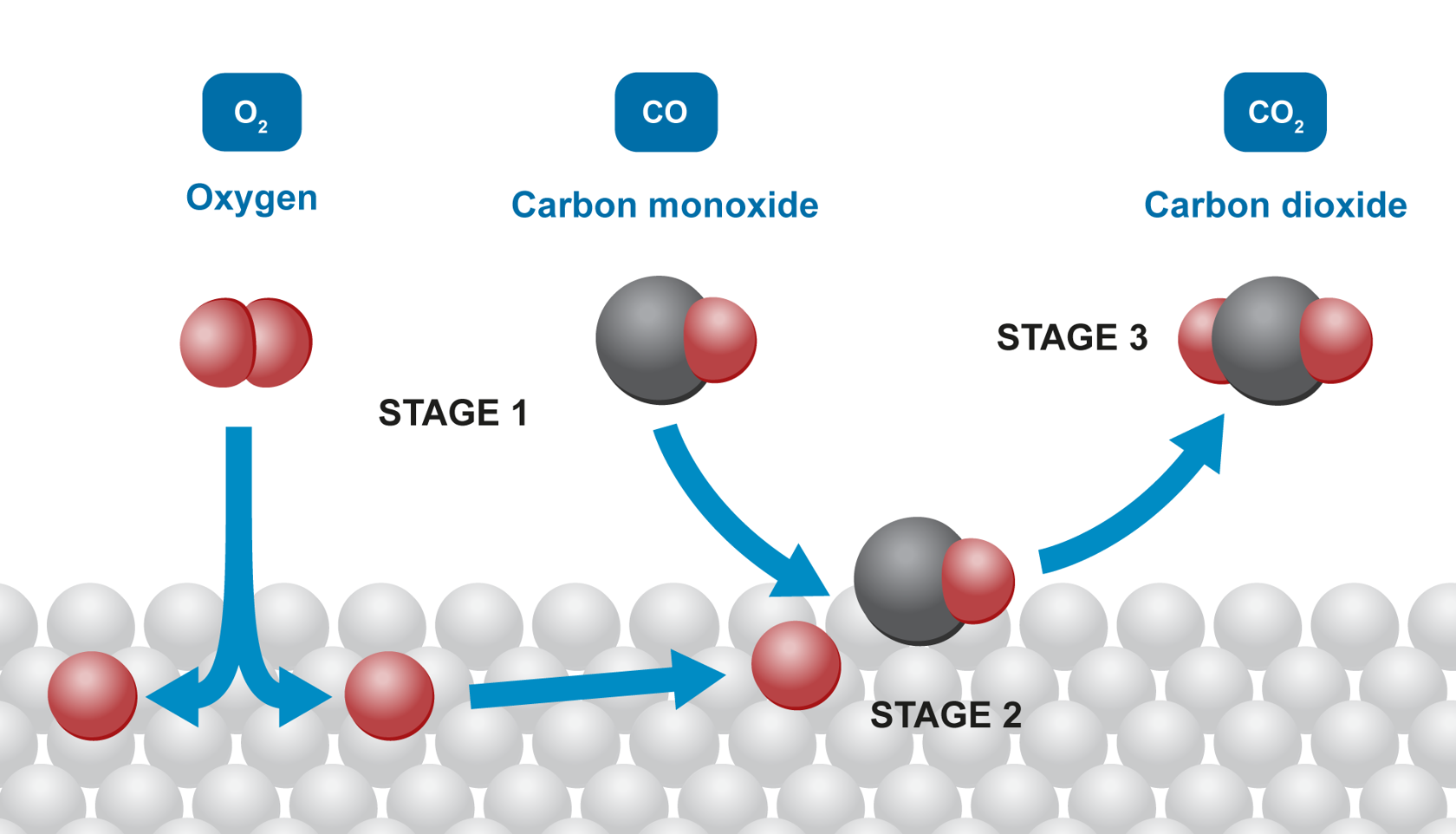
When a reaction occurs on a solid and gaseous substance’s interface, the reaction rate depends on the number of gas molecules that are attached to the surface. Put another way, the rate depends on the gas’s concentration on the surface and its proximity. The surface reaction’s rate is therefore also dependent on the gas’s pressure, which can be changed to affect the reaction rate. Only a limited number of gas molecules can attach themselves to a surface of any given size, so once the space is filled, increasing pressure does not increase the reaction rate.
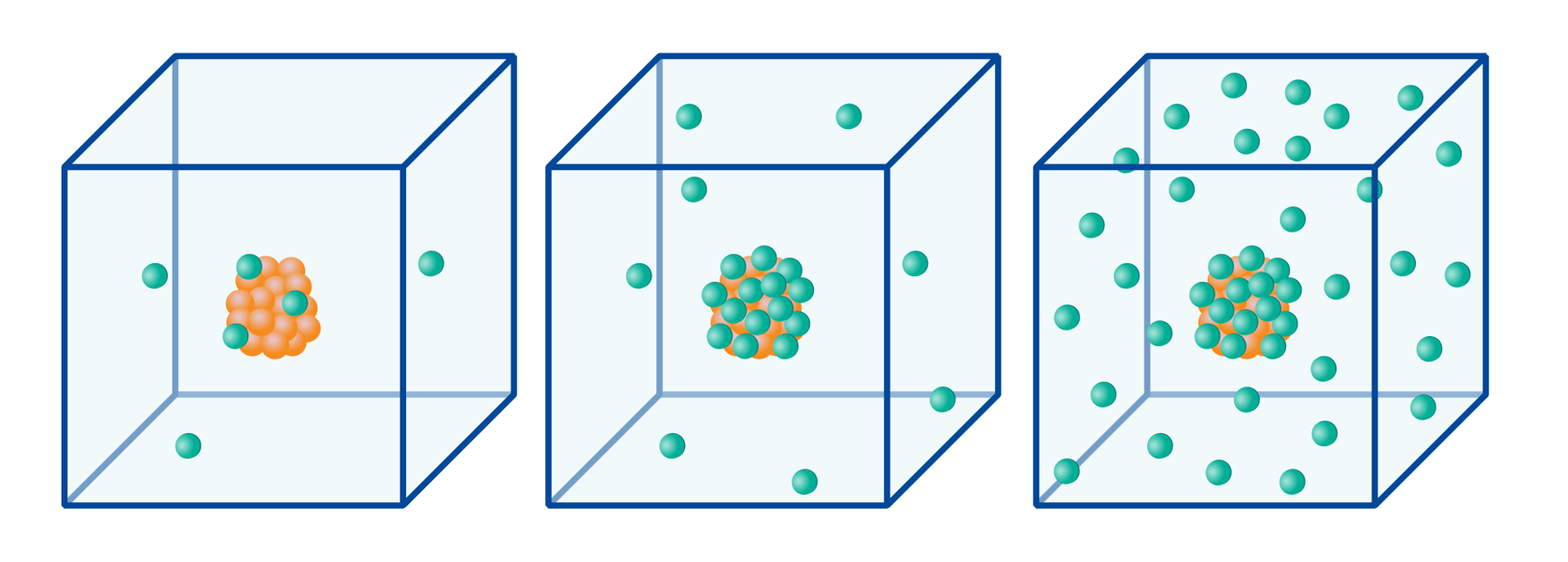
A reaction that occurs on a solid and liquid substance’s interface has a similar mechanism to a reaction on a solid and gaseous substance’s interface. One simple example of a reaction between a solid and liquid substance is when a substance dissolves into liquid. The liquid thoroughly surrounds the solid substance’s surface, and the very top layer of the solution is saturated. Once the dissolved substance diffuses away from the surface, the liquid can come into contact with the deeper layers. The further away from the surface we move, the lower the concentration is for the substance that has already dissolved into the liquid. Stirring quickly mixes the dissolved substance throughout the liquid and speeds up dissolution. When the liquid is stirred strongly, the dissolved substance has the same concentration throughout the liquid, except for a very thin layer right next to the solid substance’s surface. When a metal and acid react with each other, for example, the reaction occurs in the surface’s immediate proximity. The concentration of hydrogen ions on the surface is zero, and the concentration increases when moving from the surface to the liquid. The concentration of metal ions changes in the opposite direction.
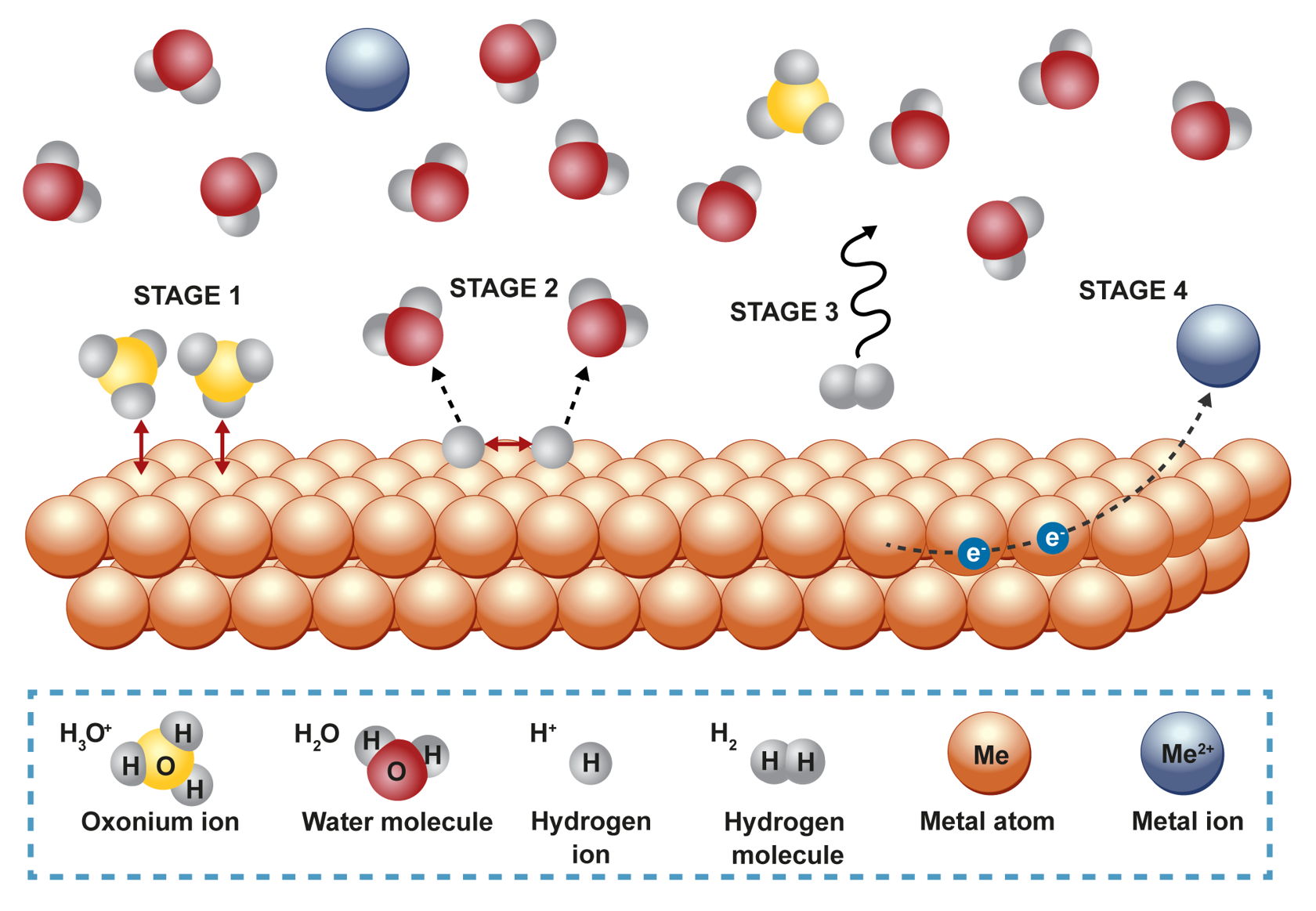
How steric hinderance affects the reaction rate
If a reacting object’s three-dimensional structure causes a steric (spatial) hinderance, it affects the reaction rate. In unimolecular reactions, the structure does not matter very much, and steric hinderance has a low impact on the reaction rate. During unimolecular reactions, practically every collision that fulfils the energy conditions results in a reaction, usually the breakdown of a certain bond in a molecule. Unimolecular reactions are important in forming ions and radicals, the denaturation of proteins and the inactivation of enzymes.
Steric hinderance has a significant impact on reaction rates for large and complex organic structures. In such structures, the reactive part of a molecule usually makes up only a small part of the entire molecule, and it may be shielded by other nearby parts. It becomes difficult for the reactive parts to come into contact with each other, which can be observed as a lowered reaction rate. Molecular vibration and rotation also disrupt the reactive parts’ ability to orient themselves in the right colliding position, which partially explains why reaction rates decrease.
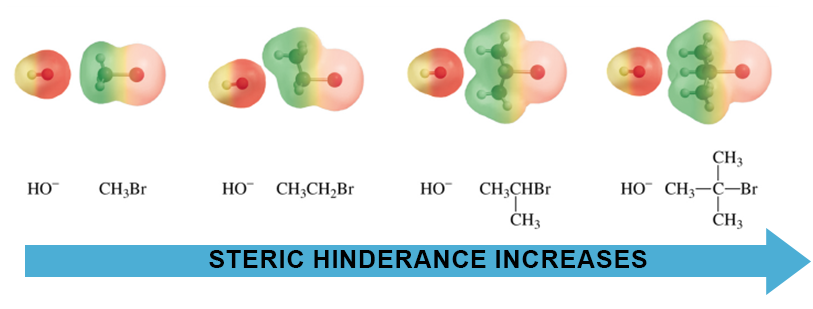
If two similar atoms collide with each other, the collision results in a reaction, as long as the collision is energetically strong enough. In cases like this, the reaction rate depends on how extensive the collision is, meaning how many collisions occur per second. The situation is almost the opposite in biochemical reactions, where collisions must happen at the exact right angle and speed and hit the reactive part of a very large biomolecule, such as a protein or amino acid. The likelihood of favourable collisions in these conditions can be up to one million times lower than the likelihood of collisions for individual atoms.
How temperature affects the reaction rate
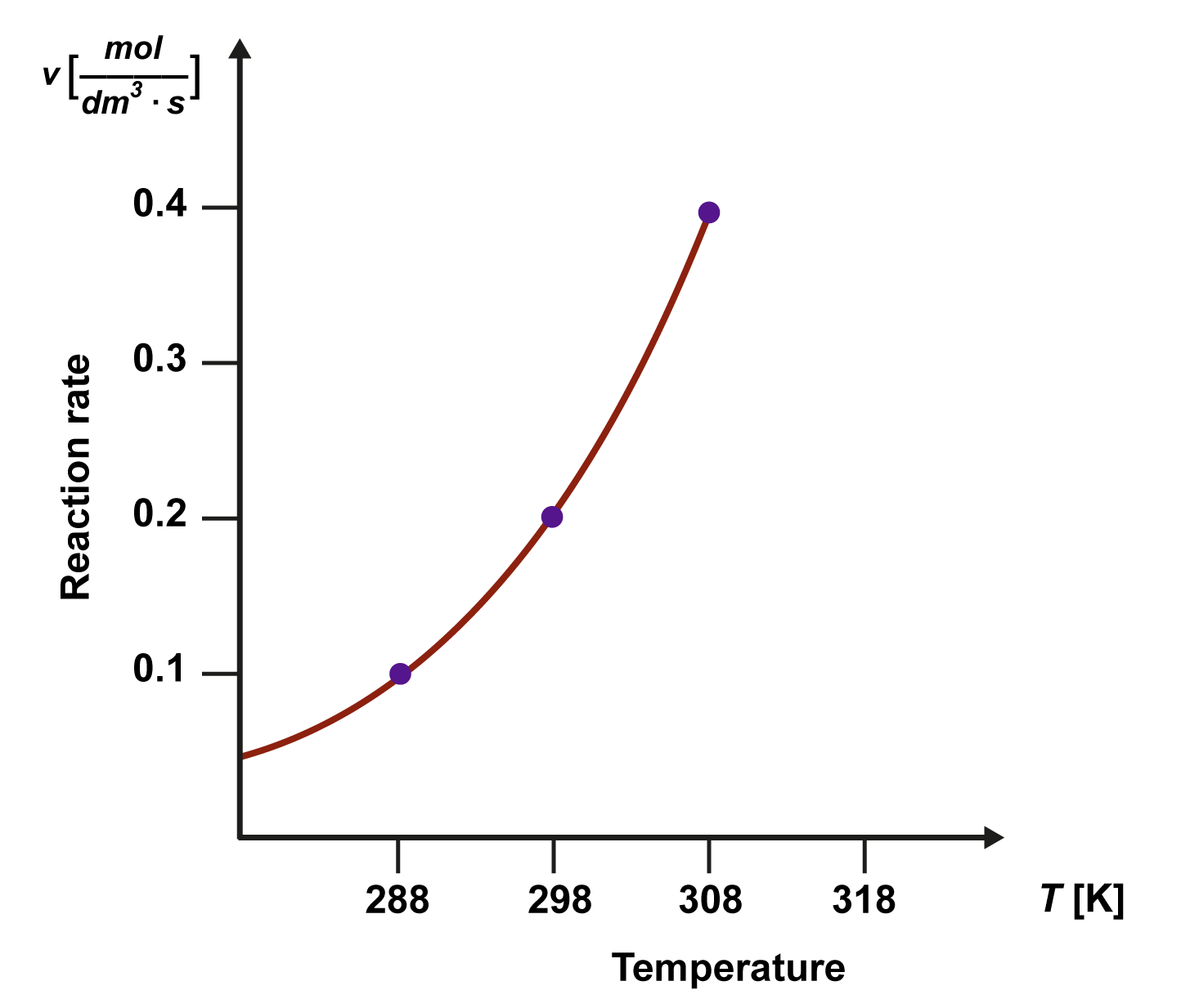
The reaction rate usually increases when the temperature rises, and the rate decreases when the temperature lowers. For example, the shelf life of food is improved with cold storage, which slows down the reactions that spoil food. At absolute zero (–273 ºC), the structural parts stop moving entirely, and no chemical reactions can occur.
At reaction conditions close to room temperature, the reaction rate doubles or triples for every 10 K increase in temperature. If a reaction also releases lots of thermal energy, there is always the danger that the reaction mixture heats too much and the reaction accelerates to an uncontrollable degree. Because an increase in temperature also increases the reaction rate, the temperature range where studies can be carried out safely is often rather narrow, only a few dozen Kelvin. If necessary, the reaction mixture is cooled, or substances that slow down reactions are added to it.
The increased reaction rate from increased temperature is explained by the increase in the kinetic energy of particles. A reaction starts once the activation energy threshold, Ea, is exceeded. At higher temperatures, there are more collisions with a high enough energy, and a larger portion of reactions results in the formation of reaction products.
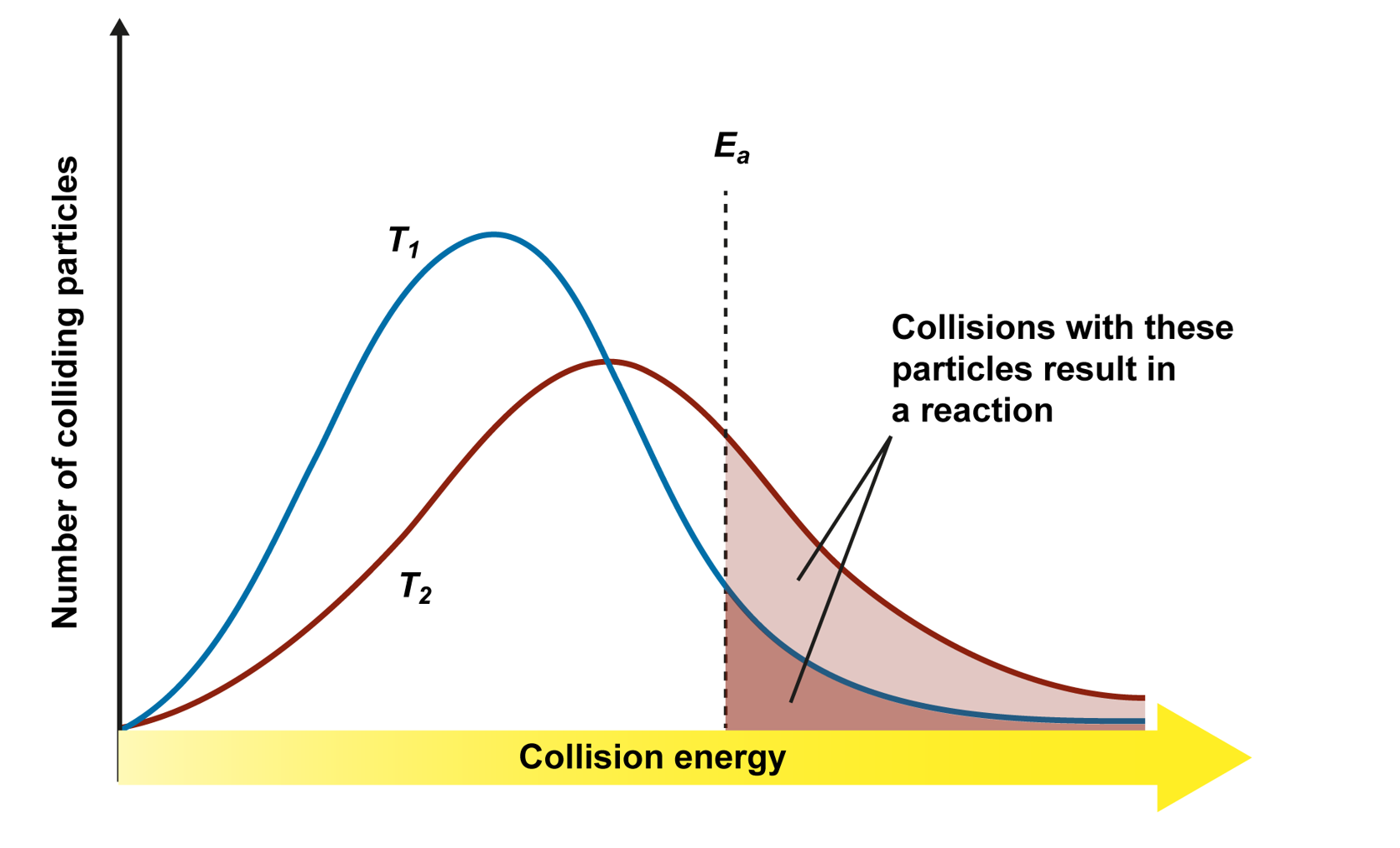
When it comes to the effects of temperature, the increased kinetic energy of particles is a more important factor than the increased number of collisions. The number of collisions does increase as the temperature rises, but the impact it has on the reaction rate is only about a thousandth of the impact that kinetic energy has.
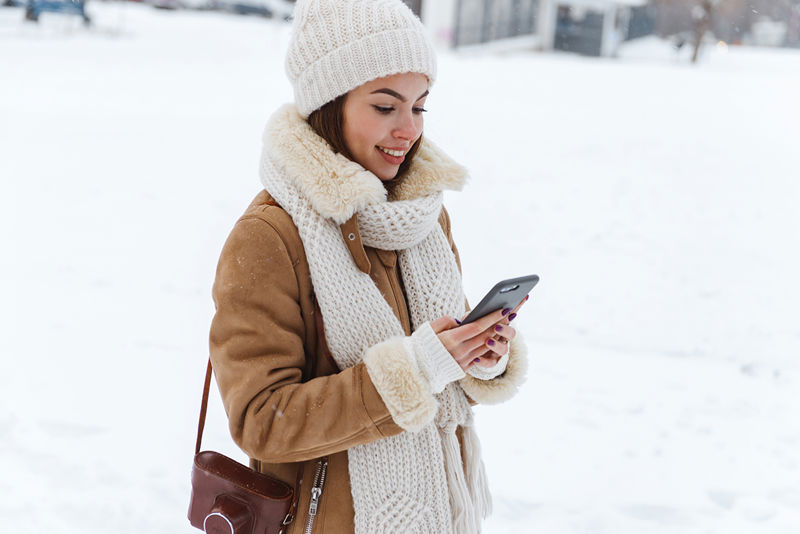
How concentration affects the reaction rate
A strong acid solution is more dangerous and corrosive than a mild one. Airborne pollutants similarly cause more respiratory problems in areas with a high pollutant concentration. In liquid and gaseous phases, concentration is directly connected to the rate of chemical reactions. For the gaseous phase, the phenomenon is studied through pressure and how it affects the reaction rate. It is also possible for concentration to have next to no effect on the reaction rate.
How concentration affects the reaction rate varies from one reaction type to the other. Doubling the concentration can also double the reaction rate, but the cause-and-effect relation is often more complicated. You cannot use an increase in concentration to directly deduce how much faster a reaction becomes. Doubling the concentration might double the reaction rate, but the rate can also become ten times greater.
For example, zinc pellets react more quickly in a strong hydrochloric acid solution compared to a mild solution, and a strong hydrogen peroxide solution decomposes into water and oxygen more quickly than a mild solution. In the same way, sodium thiosulphate and hydrochloric acid form a yellow precipitate more quickly if the used hydrochloric acid solution is stronger rather than milder. The explanation for the increased reaction rate in these cases is that there are more collisions which form reaction products in strong solutions. This happens regardless of whether the reactants are a solid and a liquid substance or two liquid substances.
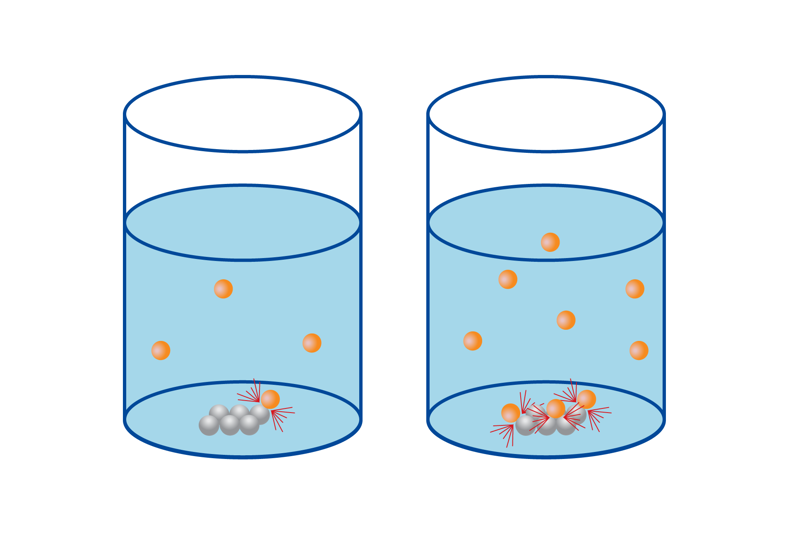
The determining factor in reactions that are based on the decomposition reaction of an individual particle is the number of particle collisions. All collisions that exceed the activation energy threshold, Ea, result in a reaction. Imagine a situation where one collision out of a million results in a chemical reaction. A solution with 100 million particles fulfils the energy requirement after 100 collisions, causing a reaction. In a reaction like this, doubling the solution’s concentration from 100 to 200 million also doubles the reaction rate.
Concentration does not always affect the reaction rate. This is true when a reaction is catalysed, for example, and the catalyst has already sped up the reaction to its maximum rate. The reaction usually occurs on the catalyst’s surface, and if the surface is entirely coated, increasing the reactant concentrations does nothing. Increasing the concentration is also not always beneficial in multi-stage reactions. In a multi-stage reaction, each intermediate reaction has its own reaction rate. Some reactions progress quickly, others more slowly. Take a two-stage reaction where reactants A and B form reaction products C and D, for example.
The reaction rate is determined by how quickly reactant A decomposes into reaction products C and D. This is called the rate-determining step. The reaction rate can be increased by increasing reactant A’s concentration. Increasing the concentration of reactant B does not yield the same benefit. Even if we increase reactant B’s concentration, the necessary C reactant is not formed any faster than before, so the overall reaction rate does not change. This kind of mechanism is typical for many organic compound reactions that involve two or more stages. The reaction is usually a tertiary haloalkane reaction that includes a varying number of other compounds, such as hydroxide ions. The reaction commonly progresses as a nucleophilic substitution reaction, where one of the reaction stages is slow and the others are faster.
The effect that concentration has on the reaction rate can be studied with the reaction rate graph. The reactant’s concentration and the reaction rate are at their highest at the beginning of the reaction. As the reaction progresses, the reactant’s concentration decreases, and so does the reaction rate.
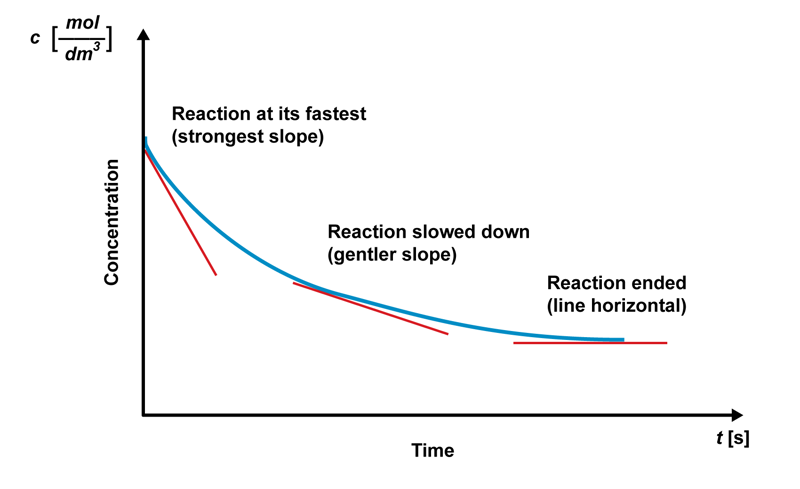
The reaction rate graphs can also be used to estimate and evaluate the factors that affect the reaction rate. For example, the sharpness of the graph’s slope can be used to compare how solution strength affects the reaction rate. When using a mild solution, the reaction rate is lower, and the graph’s early slope is gentler. When using a strong solution, the reaction rate is higher, and the graph’s early slope is sharper. The reactions end at the same final concentration, but the concentration decreases much faster when using a stronger solution.
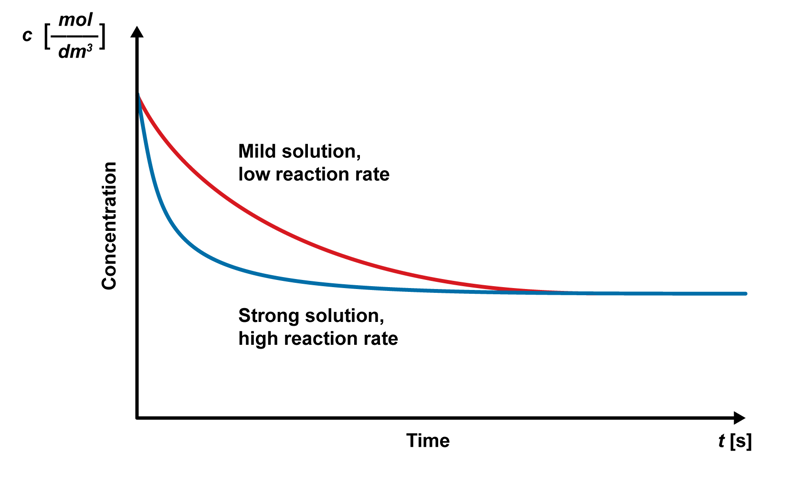
How pressure affects the reaction rate
We can affect the reaction rate of a chemical reaction by changing the pressure. This is only significant for gaseous substance reactions, because solid substances and solutions are almost impossible to compress. The pressure can be changed by changing the concentration or volume. According to the ideal gas law, changing the pressure has the same impact as changing the concentration.
, where = pressure, = volume, = amount of substance, = gas constant, = temperature
Rearrange the terms to get
Place into the equation to get
The equation’s term is constant if the temperature is not changed ( always has a constant value). Therefore, the pressure is directly proportional to the concentration. If the pressure is doubled, the concentration also doubles.
If we want to change the pressure by changing the concentration, we can add or remove an amount of reacting gas. Increasing the concentration increases the reaction rate, because the number of collisions between particles increases. Similarly, decreasing the concentration decreases the reaction rate, because there are fewer collisions between particles.
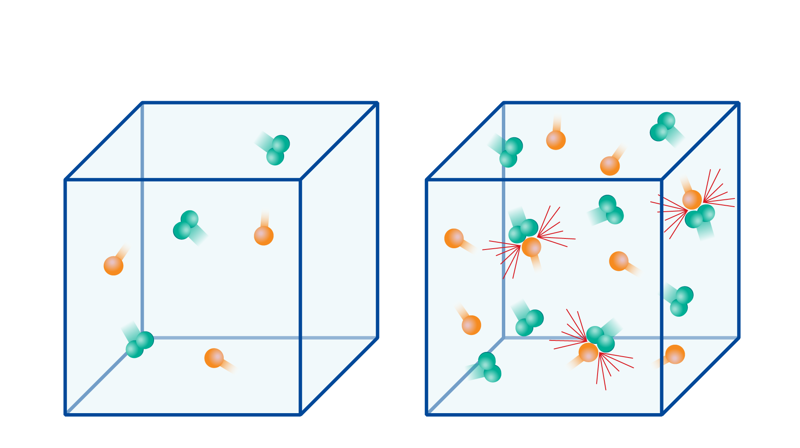
Increasing the pressure by adding or removing a non-reactive gas does not affect the reaction rate, as long as the system’s volume does not change. The reacting substances will have the same concentrations, and even though the total pressure increases, the reacting substances maintain their concentrations and partial pressures.
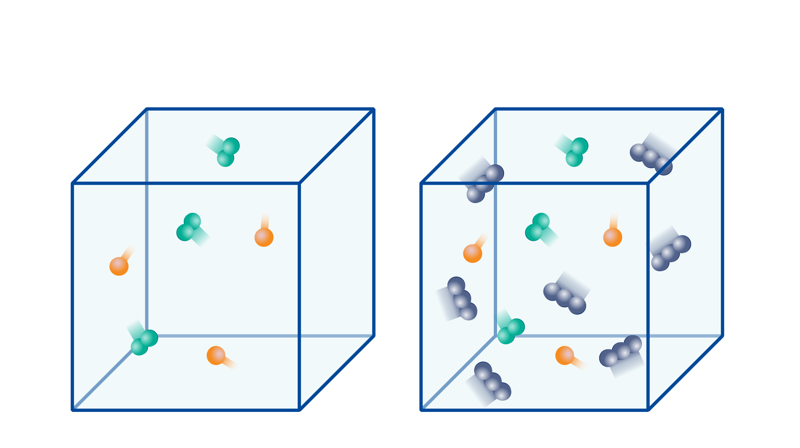
Changing the pressure by changing the reaction container’s volume alters the substances’ concentrations. As the volume increases, the reacting substances’ concentrations decrease and the reaction rate also decreases, because there are fewer collisions between particles. By contrast, decreasing the volume increases the reacting substances’ concentrations and also increases the reaction rate, because there are more collisions between particles.
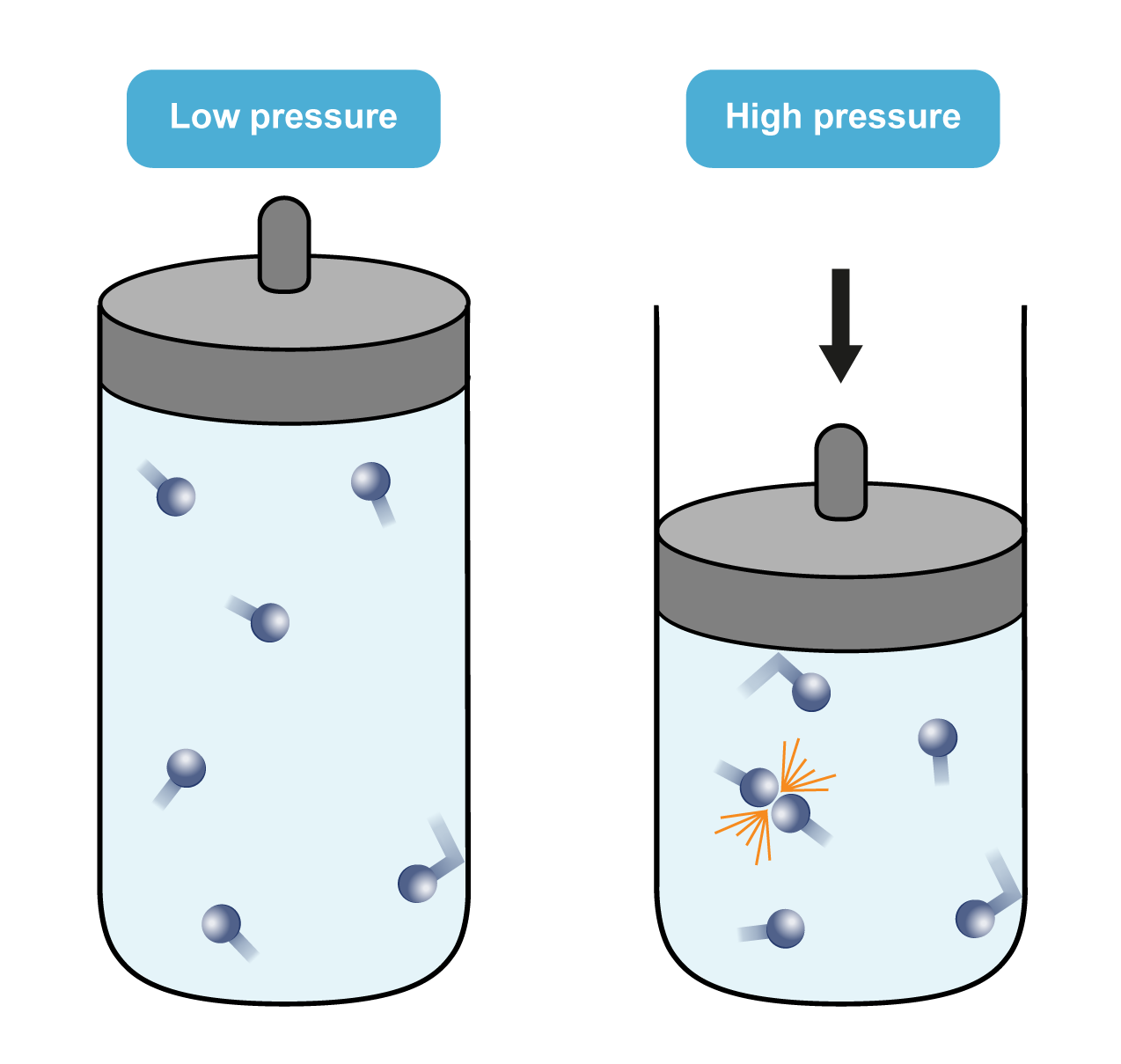
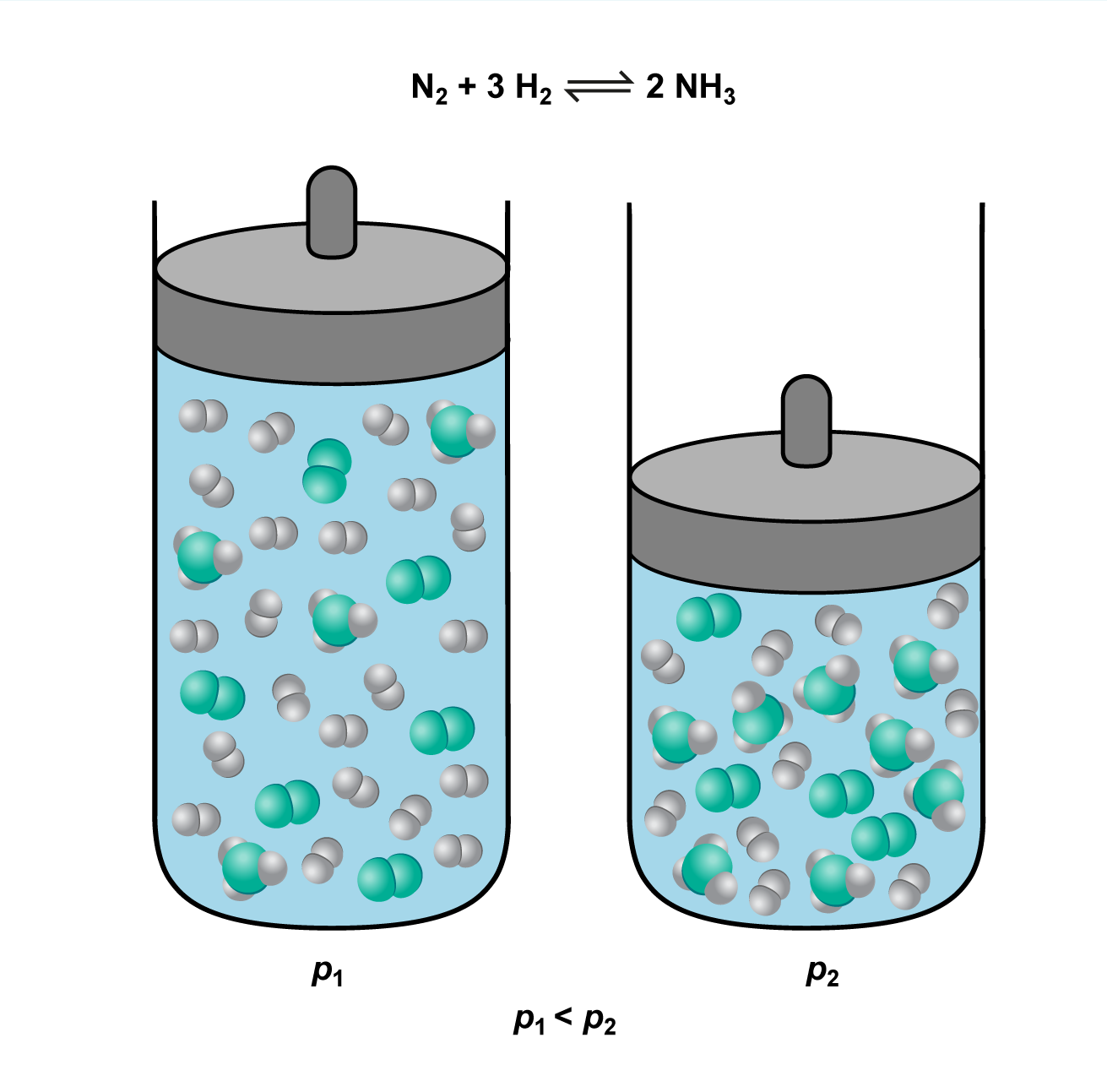
Changes in pressure are particularly important if the reaction equation’s two sides have different amounts of gaseous substances. Changing the pressure can force the reaction to progress in a pre-determined direction.
How catalysts affect the reaction rate
The Swedish chemist Jöns Jacob Berzelius (1779–1848) discovered that many reactions require the presence of another substance that is not directly part of the reaction. Berzelius named the phenomenon catalysis (from Greek, dissolution) in 1835. A catalyst generally refers to a substance that increases the reaction rate but whose amount in the reaction does not change. A catalyst that slows down the reaction rate is called an inhibitor. The phenomenon is called catalysis and the technical device where the catalysis takes place is called a catalyser. Biological catalysts are called enzymes. Catalysis is very important in nature, laboratories and industry.
Even a small amount of a catalyst can cause large substance amounts to react, because the catalyst itself is not consumed in the reaction. The catalyst’s ability to increase the reaction rate is based on the fact that it steers the overall reaction to occur through reactions that have a lower activation energy, Ea. A catalyst does not lower the activation energy. Instead, it causes other reactions to occur, and their activation energy threshold is lower than the activation energy of an uncatalysed reaction. There are two types of reactions that occur in a reaction mixture containing a catalyst: catalysed reactions and uncatalysed reactions. The proportion of uncatalysed reactions is usually clearly lower than the number of catalysed reactions. However, a catalyst cannot cause reactions that would not otherwise occur.
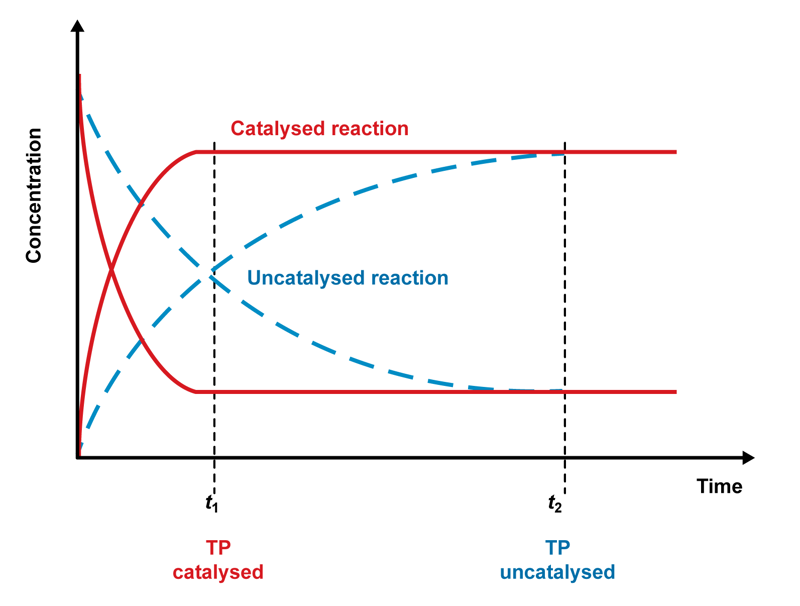
Catalysts are often reaction-specific, meaning that they only speed up or slow down specific reactions. By choosing the right catalysts, we can speed up or slow down particular reactions among parallel reactions. Reaction specificity is especially characteristic for enzymes. It is also common for a mixture of two catalyst substances to be more effective than either catalyst would be on its own. The efficiency of catalysis and the reaction rate can be increased by adding a small amount of another catalyst into a mixture. For example, the primary catalyst in ammonia synthesis is iron, but the reaction rate can be further increased by adding in a small amount of potassium and aluminium oxides.
How a substance’s total area affects the reaction rate
It is difficult to ignite a whole log, but chopping it into small pieces makes ignition much easier. Very fine wood dust can combust explosively quickly. A sugar cube dissolves slowly in water, but stirring the liquid speeds up the dissolution significantly.
Based on these observations, we can deduce that the larger a reacting substance’s area is, the higher the chemical reaction rate is. The same is true for stirring. Stirring increases the reaction area, makes it easier for reacting substances to come into contact with each other and increases the reaction rate. Then again, a pile of fine wood dust does not ignite very easily, because oxygen cannot come into contact with the wood material inside the pile. Instead, a big pile of wood dust ignites almost as poorly as a whole log. This can be proven by comparing the ignition of magnesium powder and magnesium thread, for example. It almost seems like the thread ignites more easily than piled-up powder. However, airborne wood or magnesium dust ignites quickly.
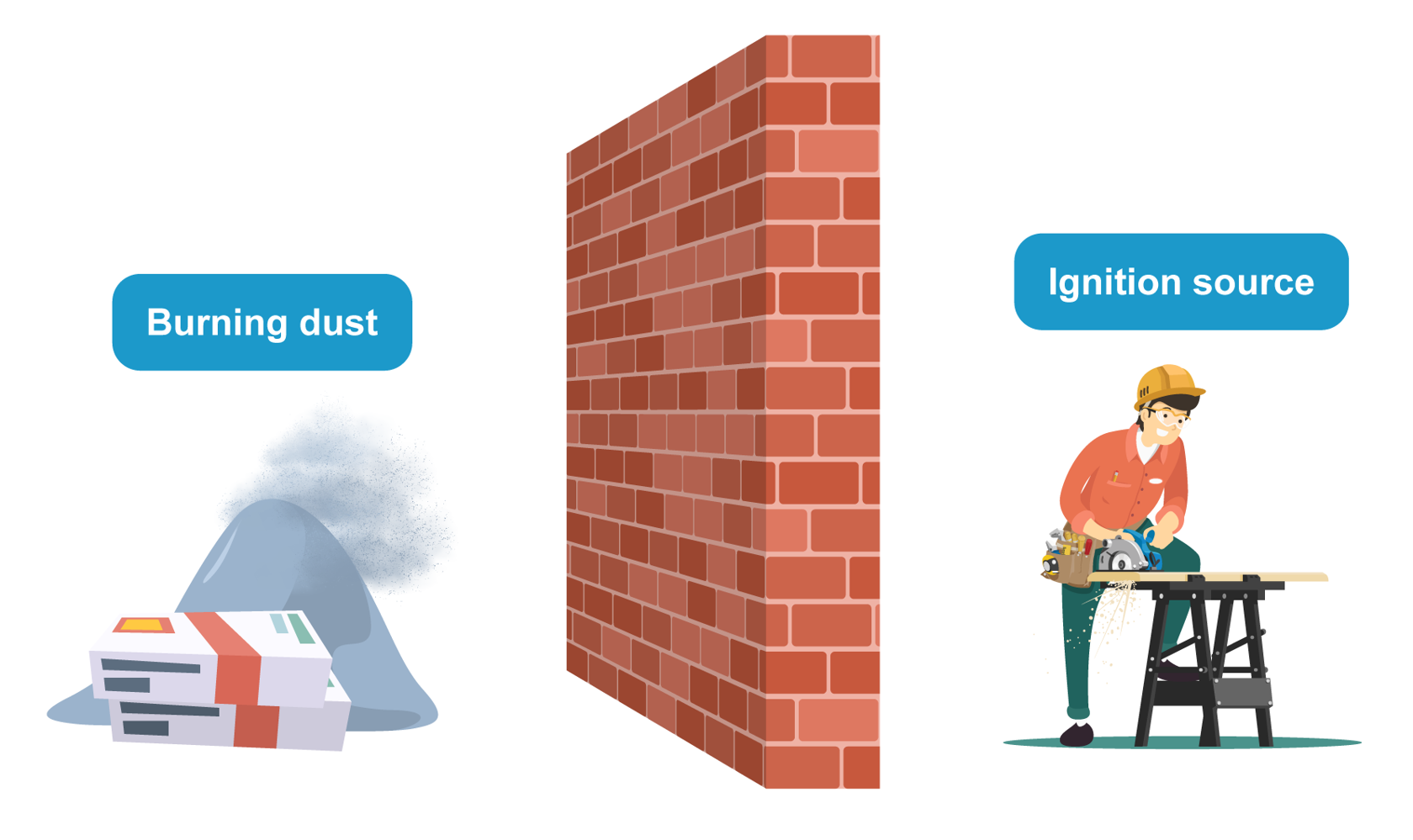
Calcium carbonate can be made to react with hydrochloric acid more quickly by using powder instead of pellets. The decomposition of hydrogen peroxide into water and oxygen can also be made faster if the reaction is catalysed with manganese oxide powder instead of manganese oxide pebbles. A car’s catalytic converter makes exhaust gases less harmful with catalysts. The exhaust gases are in the catalytic converter for only a moment, so the reactions must happen very quickly. The catalysts are usually platinum, palladium and rhodium, which are very expensive metals. The catalytic converter has a honeycomb-like structure, where the ceramic surface has a very thin layer of catalyst attached to it. Gases flow by the surface, causing substances like carbon monoxide and nitric oxide to react and form carbon dioxide and nitrogen.
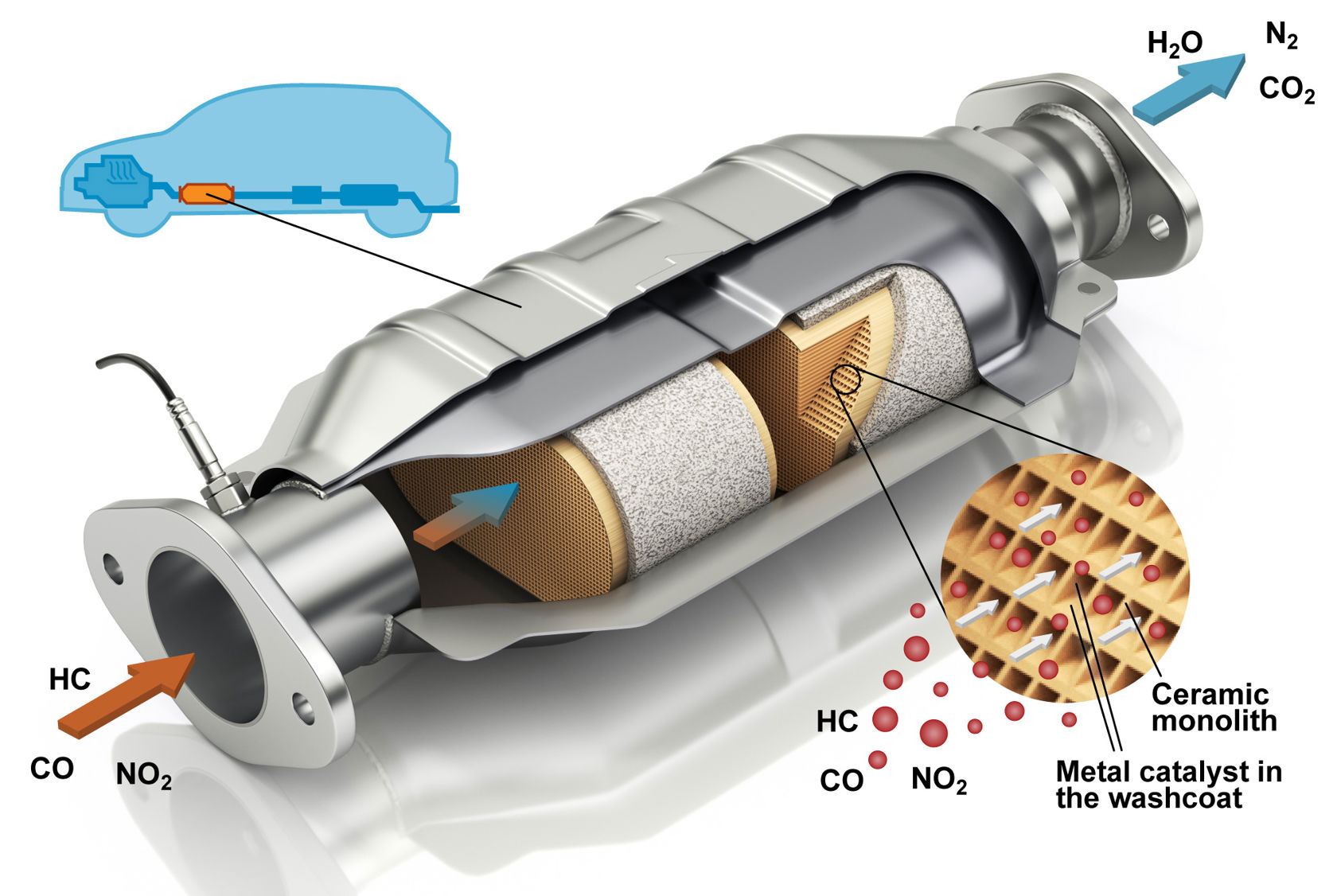
A substance’s total area affects the reaction rate due to the increase in favourable collisions. Increasing the reaction area increases the likelihood of collisions that result in the formation of reaction products. We can look at the reaction between magnesium and hydrochloric acid as an example. The reaction occurs between the metal atoms and oxonium ions on the surface of metallic magnesium. If the pellet size is big, the reaction only occurs on the pellet’s surface, not inside it. Chopping the pellet into smaller pieces increases the reaction area, and the reaction occurs more quickly.
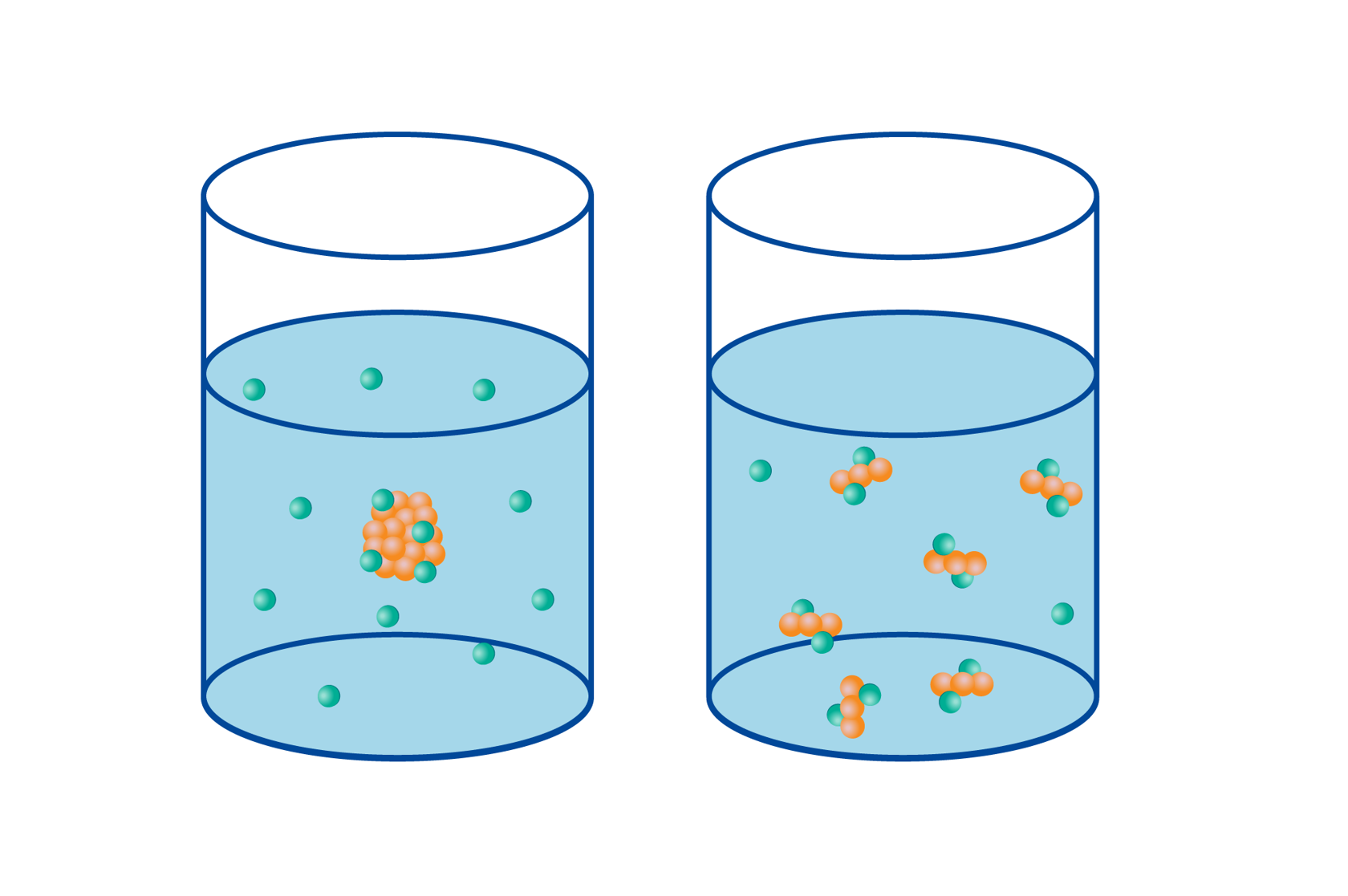
The total area is especially significant when one of the substances in a reaction (a reactant or a catalyst) is in its solid phase. In such a case, the reaction mostly occurs on the solid substance’s surface as a surface reaction. When all the reacting substances are in the same phase as either liquids or gases, the substances are able mix better, and the area has a smaller effect.